CMDR-DOS: Commodore DOS on FAT32
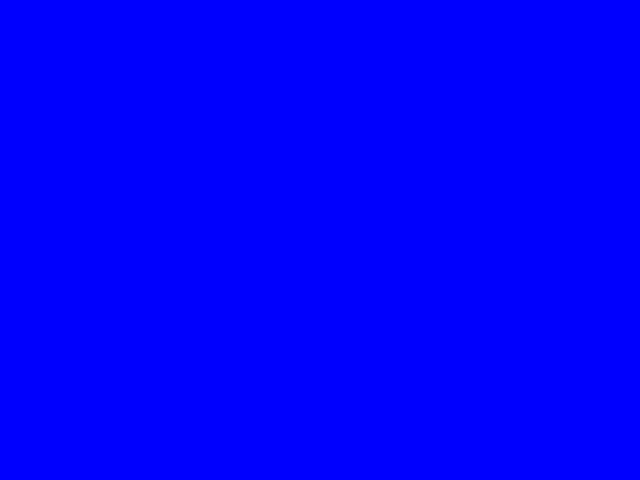
All disk drives connected to the Serial Bus of a Commodore 64 speak the Commodore DOS protocol, from the popular 1541 5.25″ drive to the modern sd2iec SD card interfaces. CMDR-DOS is a new and open source implementation of the Commodore DOS protocol, using SD cards with the FAT32 filesystem and supporting advances features like partitions, subdirectories and timestamps – and running on a 65c02!
FAT32 Filesystem for the 65c02
We are presenting the (to our knowledge) first full-featured open source library for 65c02 CPUs for accessing FAT32 formatted disks.
Using the OS X 10.10 Hypervisor Framework: A Simple DOS Emulator
Since Version 10.10 (Yosemite), OS X contains Hypervisor.framework, which provides a thin user mode abstraction of the Intel VT features. It enables apps to use virtualization without the need of a kernel extension (KEXT) – which makes them compatible with the OS X App Store guidelines.
This is Copyright 1983 Microsoft – NOT!
If you look at a hexdump of any version of the Logitech mouse driver for MS-DOS, you will see the following:
Reverse-Engineering DOS 1.0 – Part 2: IBMBIO.COM
Update: The source is available at github.com/mist64/msdos1
Reverse-Engineering DOS 1.0 – Part 1: The Boot Sector
Update: The source is available at github.com/mist64/msdos1